Hydrolysis Rovira
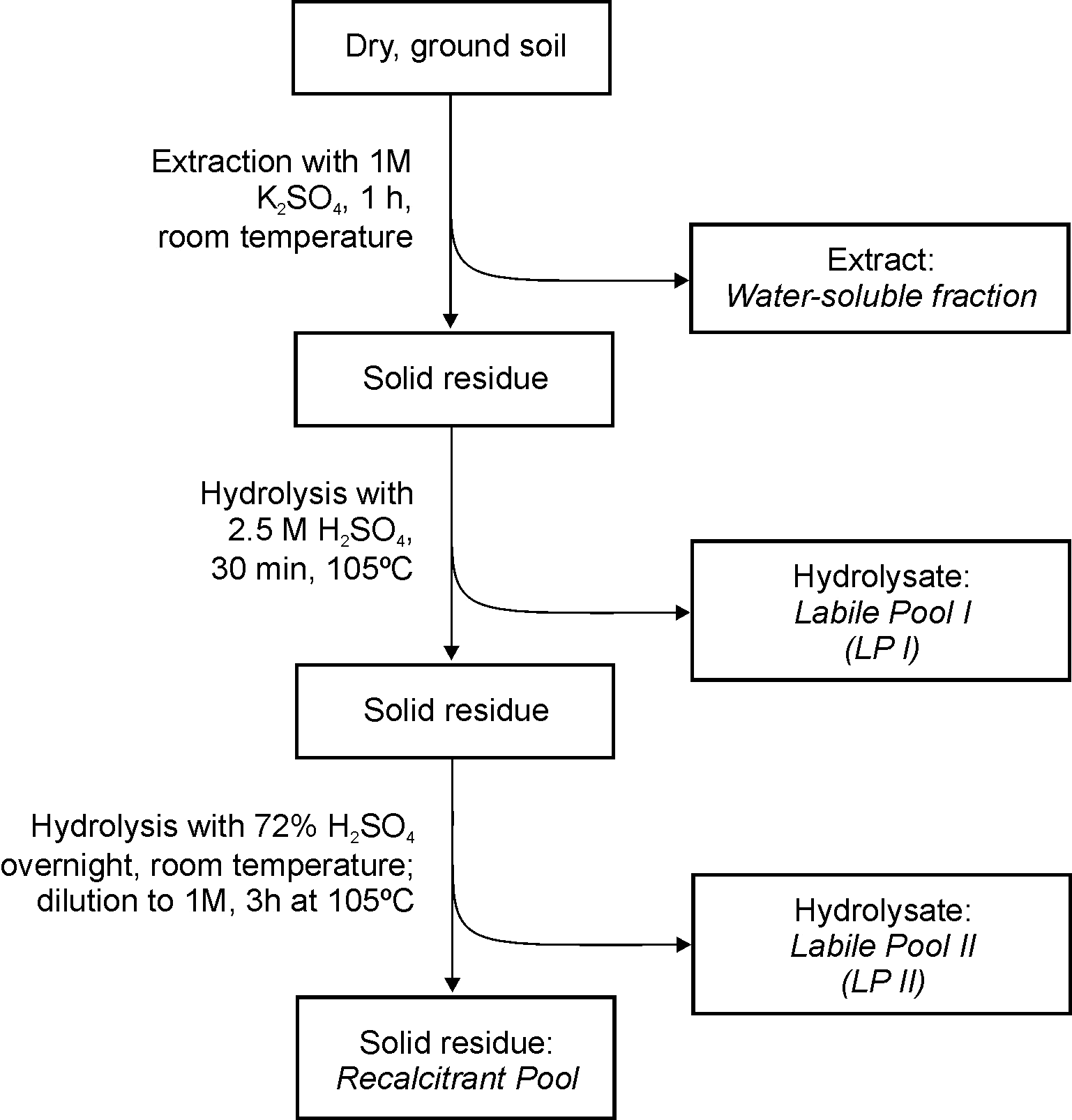
Description and working steps
a) Hydrosolubles
Put 0.5 g of finely ground soil in a pyrex tube (take note of the exact initial weight with 0.1 mg precision). Add 20 ml of 0.5 M potassium sulfate. Seal the tube, and put it in the block digestor, 1 hour at 105 ºC. Remove the tube. Let cool. Transfer the liquid to a centrifuge tube. Centrifuge at the speed needed for a complete removing of fine mineral matter (silt and clay; the liquid phase may retain small suspended organic particles). Transfer the liquid phase to a beaker. Wash the original pyrex tube with water, transfer the washings to the centrifuge tube and centrifuge again. Add this new washing to the previous one. Transfer the liquid to a volumetric flask (50 mL if possible, 100 mL otherwise), through a filter if necessary (to remove the floating organic debris). Made up to the mark. This fraction is labelled as the water-soluble pool (WS).
Recover the organic debris retained in the filter, and return them to the residue of centrifugation, which contains the non-extracted material. Dry the residue (50 ºC), and transfer it quantitatively to the Pyrex tube, for the next step.
b) First hydrolysate
Add to the pyrex tube 20 ml of 2.5 M H2SO4. Seal the tube, and put it in the block digestor, exactly 30 minutes at 105 ºC. As for the rest, operate as in the previous extract (hydrosolubles), including the final step of drying the unextracted residue and transfer it to the pyrex tube for the next step. This new fraction is labelled as Labile Pool 1 (LP1).
c) Second hydrolysate
Add to the pyrex tube (containing the material remaining from the previous extraction) 2 ml of 13 M H2SO4. Shake overnight, in an end-over-end shaker (tubes in vertical position; the aim is to facilitate acid penetration throughout the whole sample). No strong agitation is needed, for all the sample should be maintained at the bottom of the tube, covered by a thin layer of acid. Next morning, add to the tube 24 ml of water, to obtain a final acid concentration of about 1 M. Water addition should be done in a single step: the rapid mixing and homogenization will prevent an excessive heating (as expected when water is added to concentrated sulfuric acid). Homogenize the suspension, seal the tube, and put it in the digestor, at 105 ºC for 3 hours. As for the rest, operate as in the previous extracts. This new liquid fraction is labelled as Labile Pool 2 (LP2).
d) Residue
The final residue, dry, is transferred quantitatively to a pre-weighed beaker, dried at 60 ºC to constant weight, and weighed. This residue is the Recalcitrant Pool (RcP).
e) H2O2 oxidation
Important note: this step was not performed in the ‚Ring Experiment‘.
A subsample of the recalcitrant pool (200 mg, or less if the amount of recalcitrant pool is very small) is put in a Pyrex tube. Take note of the exact initial weight. Add 100 ml of 10% H2O2, and put the tube in the digestor, open (not sealed), at 50 ºC overnight. Next day, transfer the content of the tube to a centrifugue tube, and recover the residue by centrifugation. Discard the liquid. Transfer again the residue to the same pyrex tube. Repeat the oxidation process twice (i.e., three oxidations in total). The remaining residue is finally transferred to a pre-weighed beaker, dried at 60 ºC to constant weight, and weighed. This residue is the Refractory Pool (RfP). Note that this Refractory Pool is meant to be a part of the Recalcitrant Pool (RcP). Of course it is possible to obtain from both a 'recalcitrant but not refractory pool' as the difference between both.
f) Analyses
All pools must be analized for carbon and, if desired, also for nitrogen. The solid pools (RcP and RfP) may be analized easily using an elementar analyzer (ThermoQuest, Leco or similar). The liquid pools are not so easy to analyze for carbon, because usual TOC devices often run poorly on liquids rich in salts or in acid hydrolysates. Dichromate oxidation methods are the choice in such a case.
In the liquid fractions, analyses of carbohydrates, phenolics and so on are of obvious interest. The absorption at 254 or 280 nm is often taken as a measure of the abundance of aromatic carbon: in such a case it is recommended to pass the extracts previously trough a cationic resin, to remove iron, which interferes in this analysis (this is particularly important in LP1). The decationized extracts may be studied by liquid-state 13C NMR to obtain a panoramic view of their composition.
Initial Aim
As in all chemical fractionation methods, the ultimate aim is to submit the sample to a series of extractions of increasing strength. The underlying concept in the whole protocol is the Saeman's hydrolysis for obtaining cellulose: the third step (to obtain LP2 and RcC) is essentially the same process used to obtain Klason lignin from lignocellulose. The ultimate source for our protocol is the classic paper of Oades et al. (1970), whose lecture is recommended, and from which we proposed the two-step sequential hydrolysis with 2.5 M and 13 M sulfuric acid.
Acid hydrolysis has been often used for soil organic matter fractionation, even though HCl is most often used, instead of H2SO4. But in such a case cellulose would be not (or only partly) hydrolyzed, and then cellulose would be included in the recalcitrant pool (RcP). Thus the exact meaning of RcP would change substantially. On the other hand the HCl hydrolysates can not be analyzed by dichromate oxidation, owing to the interference of chloride.
The above protocol follows that given in Rovira et al. (2012), which is an extension of the original protocol given in Rovira & Vallejo (2002). A slight modification has been applied, though. In the original method (2012) the extraction of water-soluble compounds was performed by agitation at room temperature, while in the current protocol it is performed by heating at 105 ºC. The reason is that the amount of WS organic matter extracted at room temperature is often not enough for many analyses. Performing the extraction at high temperature implies that the meaning of the WS extract changes a bit: it involves not only the organic matter soluble at a given moment, but also the organic matter that could become soluble in a medium or long-term. At any rate, organic matter that can be extracted without acid hydrolysis. Of course the increased relevance of the WS pool results in a decreased relevance of the next one (LP1).
Advantages
Simplicity and efficiency. At first glance, the protocol is not too simple: it involves several steps, none of them particularly difficult but all needing care. Nevertheless, the protocol is easily applied to long series of samples: it depends on the capacity of the aluminum block digestor. Block digestors of 40 or more places are common, and thus the study of long sample series is highly feasible. The time needed for a single soil sample is much lower than in other methods than must be applied to the soil samples one by one. The method is highly efficient.
The above protocol may be easily simplified by supressing the two extremes (WS pool and Refractory Pool). In such a case, we would go in a natural way to the original acid hydrolysis protocol, given in Rovira & Vallejo (2002, 2007).
Disadvantages
The protocol reflects the chemical composition of organic matter, whereas current knowledge says that the physical protection of soil organic matter is the main constraint for its stability. Thus the simple distribution of organic matter between labile and recalcitrant pools, as they are defined in this protocol, does not give information about its stability, as we already observed (Rovira et al. 2010). Labile (hydrolyzable) and recalcitrant (unhydrolyzable) organic matter are found in both fast-cycling and slow-cycling pools. The method gives an overall view about the composition of soil organic matter, not (or not directly) about its stability.
Owing to this drawback, it is recommended to apply this method to the physical fractions obtained by density- or size-fractionation methods. In this way it helps to give a very complete picture of the characteristics of soil organic matter, in which physical protection and biochemical quality are combined. See Rovira & Vallejo (2007) and Rovira et al. (2010) for examples of this approach.
References
Oades J.M., Kirkman M.A., Wagner G.H. (1970). The use of gas-liquid chromatography for the determination of sugars extracted from soil by sulfuric acid. Soil Science Society of America Proceedings 34, 230-235.
Rovira P., Jorba M., Romanyà J. (2010). Active and passive organic matter fractions in Mediterranean forest soils. Biology and Fertility of Soils 46, 355-369.
Rovira P., Romanyà J., Duguy B. (2012). Long-term effects of wildfires on the biochemical quality of soil organic matter: A study on Mediterranean shrublands. Geoderma 179-180, 9-19.
Rovira P., Vallejo V.R. (2002). Labile and recalcitrant pools of carbon and nitrogen in organic matter decomposing at different depths in soil: an acid hydrolysis approach. Geoderma 107, 109-141.
Rovira P., Vallejo V.R. (2007). Labile, recalcitrant and inert organic matter in Mediterranean forest soils. Soil Biology & Biochemistry 39, 202-215.